What Else Are You Supposed to Test On?
Modern research methods without animal testing
I. Introduction
It is claimed time and again that animal experiments are necessary to ensure safety for humans and that without animal experiments medical progress would come to a standstill. Numerous scientific publications show that this is not true (1–4). Criticism is voiced not only by animal rights organisations, but also by numerous renowned researchers from around the world, as well as by institutions of the European Commission and international political bodies. More and more countries, including the United States (5), the Netherlands (6), Great Britain (7) and Norway (8), are developing concrete strategic plans to at least partially phase out animal testing. The main problem is that the predictive power of animal experiments is not reliable with regard to questions relevant to humans. Transferring the results to humans poses an incalculable risk.
There are, however, numerous animal-free in vitro methods today that are more reliable and cost-effective than animal experiments and, unlike animal experiments, deliver test results relevant to humans. However, in order to bring about a paradigm shift, animal testing must be abolished as the “gold standard” in research, and research funds must be reallocated. In Germany, animal experimentation projects are still funded with billions of taxpayers’ money every year, while modern, animal-free research receives only millions of dollars (9).
This brochure provides an overview of innovative animal-free research methods and explains why they are scientifically superior to animal experiments.
Nowadays, there is such an abundance of excellent cruelty-free research methods that it is hard to keep track of them. Every day there are numerous reports about new innovative procedures being developed by researchers around the world. In order to facilitate an overview and a targeted search for such procedures, we have developed the NAT Database, a free bilingual online database (German and English) for animal-free research methods (www.nat-database.de). NAT stands for Non-Animal Technologies. The database contains hundreds of entries and is continuously expanding. The entries contain a short summary of the method, including sources, and information about the responsible researcher and the corresponding research institute or company. The search mask allows a free keyword search as well as filtering options by subject area (e.g. oncology or toxicology), model (e.g. multi-organ chips), country etc.
II. Innovative Research Models in the 21st century
Today’s in vitro models used for biomedical research are more complex and physiologically correct than ever. They range from three-dimensional cell cultures to organoids and multi-organ chips, on which several organ models are combined and connected with simulated blood circulation and urinary system. These human cell models can be grown from induced pluripotent stem cells (iPSCs), cells that can be differentiated into any cell type, for instance nerve or skin cells. The cells can come from both healthy donors and patients. They are particularly pertinent as disease models and for developing drugs or testing toxins. Many of these systems have already been successfully validated, especially in the area of statutory animal testing such as chemical safety testing. Ongoing optimisation, such as the integration of blood vessels and the immune system, means that the human-based in vitro models can overcome the problems posed by the lack of transferability of animal experiments to humans.
Establishing these systems and developing complex computer simulations (in silico methods) plays a decisive role in the scientific discussion about the abolition of animal experiments. These methods offer research models that are already better at predicting results in humans than animal experiments, as comparative studies have shown (10–12). Combining human studies and imaging techniques provides scientific research with ideal tool for generating reliable research results.
- In vitro (Latin: “in glass”, i.e. in a test tube): test systems with pain-free substances such as cells, tissues, microorganisms, etc.
- In silico (Latin: “in silicon”): computer-based models
- In vivo (Latin: “in the living”): experiments that take place in the living organism.
The eye-on-the-chip consists of human corneal and connective tissue cells as well as a blinking eyelid. (Image source: BIOLines)
1. Organoids and 3D Cell Culture
In recent years, human-based in vitro research has made spectacular developments. Around 2010, researchers first succeeded in growing so-called organoids in the laboratory (13–15). These are three-dimensional human cell cultures that mirror the appearance and function of a human organ surprisingly well (16). To date, mainly two-dimensional cell cultures have been used in biomedical in vitro research. These comprise “immortalised” cell lines in a flat layer of cells. Such a model is a far cry from the structure and function of a three-dimensional organ. Modern three-dimensional cell culture systems such as organoids not only have numerous advantages over the familiar cell lines, but also over animal experiments. Human-based in vitro models such as organoids are essential for drug testing and research into human diseases. They also have the unique advantage of allowing personalised medicine, tailored to the human individual. Animal experiments can never have this capacity.
iPSC – All-Purpose Cells
Human organoids are grown using human stem cells (17). The stem cells are either isolated from tissue biopsies, or cells from a human donor – such as skin or hair root cells – are genetically reprogrammed to induced pluripotent stem cells (iPSC). Dental pulp or blood can also serve as a source of stem cells. The great advantage of the iPSC-based method is that obtaining donor cells is uncomplicated and painless for humans and that the iPSCs created can be used to create virtually any organoid in vitro.
Miniature Organs – Images of Human Organs
Organoids such as intestinal, cerebral, hepatic or renal organoids, are cultivated by adapting the culture conditions, especially the growth factors used. The organoids – or miniaturised organs – are complex three-dimensional structures comprising different cell types and usually half a millimetre to one millimetre in size. They can be cultivated over several weeks or months in cell culture plates and can be grown in vitro for almost indefinitely. Both iPSCs and the organoids themselves can be stored in a frozen state, with huge biobanks currently being created, containing iPSCs or organoids from several human donors (healthy and diseased) (18). This allows samples to be thawed when needed and reintroduced to culture in order to conduct the desired tests.
Organoids preserve the properties of the cell donor in vitro, making them an ideal disease model for biomedical research when generated from patients. In addition, organoids can be genetically modified in vitro in such a way that disease genes are either corrected in a targeted manner (19) or integrated (20). Such disease models deliver much more pertinent results than genetically modified “animal models”.
As all organs have their own stem cells, any organ can be used to grow an organoid in the laboratory. The different colours in fluorescence microscopy images show that the organoids have complex and diverse structures resembling those of the real organ.
Illustration: Doctors Against Animal Experiments Germany
Three-dimensional cell models such as organoids allow excellent research into both the molecular causes of human diseases and therapeutic approaches (21). Cerebral organoids (“mini brains”) or other complex neuronal cell models have already been grown with samples from patients suffering from Alzheimer’s disease. (22), Parkinson’s disease (23), schizophrenia (24), autism (25) and Huntington’s disease (26). In the case of neurological diseases such as Alzheimer’s disease, the need to establish new model systems based on human cells is particularly urgent, as research to date using hundreds of “animal models” has not facilitated satisfactory treatment. (27–29).
Human mini brains developing brain tumours. The bottom row (d-f) shows healthy brain tissue in blue and the tumours in purple. The mini-brains’ diameter is about 0.5 mm. Illustration: Plummer et al - SciRep 2019 (1).
Ideal Disease Models
Insufficient efficacy and unacceptable side effects often lead to therapies having to be discontinued or even drugs being withdrawn. Three-dimensional in vitro models grown from patients’ cells are ideally suited as a disease model, as they provide results relevant to humans. Using nerve cells cultured from iPSCs from anorexic patients, it was possible to identify a gene that appears to be associated with anorexia nervosa (30). Human brain organoids are also used for cancer research by adding human tumour cells to them so that brain tumours develop in them (31). Based on this in vitro model, active substances can then be tested with regard to their efficacy and effectiveness.
Personalised Cancer Therapy
Since every person and every tumour reacts differently to a particular treatment, it is a great advantage that iPSC-based in vitro models enable personalised medicine. This has already arrived in clinical application: In consultation with the treating oncologist, a patient’s tumour biopsy sent to the laboratory, where it is used to grow tumour organoids (“microtumours”). Various therapy options can then be tested and the most effective individual medication is finally administered to the patient (32). Microtumour technology is also suitable for research into new drugs, whose efficacy can be analysed in the tumour organoids (33,34). The development of cancer drugs in animal experiments carries the risk of results that are not transferable to humans. In light of the individually different effects of cancer drugs in humans with regard to efficacy and side effects, personalised cancer therapy has an enormously high potential as opposed to testing on other species such as mice in which human tumours are artificially grown (35).
Microtumour technology facilitates personalised cancer therapy. Tumour organoids are grown from a patient’s tumour in vitro. Subsequently, various therapeutic options are tested and the patient is finally administered the drug that presented the best effect in vitro.
3D bioprinting
Complex human cell models can not only be cultured from stem cells, but also constructed using 3D bioprinting (36). For this purpose, a 3D printer is used, with special bio-inks that contain not only a gel-like substance but also the human cells for the model. However, bio-inks often contain substances of animal origin such as fetal calf serum (see box S...). The “clean bioprinting” approach aims to establish a system completely free of animal ingredients (37). A variety of complex cell models containing different human cell types are printed using CAD/CAM and assembled in different arrangements to create an organ-like structure. Thus human heart, skin or lung models are printed to test drugs, research diseases or infectious processes, and test the toxicity of substances (38–40).
2. Like a “Mini Human”: Multi-Organ Chips
One in vitro system that is of the greatest significance for the future of biomedicine and drug development is the so-called multi-organ chip (MOC) (41). In MOCs, multiple organoids or similar human cell models are mounted on a biochip, connected to each other via a microchannel system. The microchannels that connect the organs on the chip simulate blood circulation and, if there is a kidney model on the chip, urinary circulation. Samples can be taken from the microchannel system, which correspond to a blood sample or urine sample taken from the patient. The organs on the chip have a metabolism and can interact with each other and exchange substances via the microchannel system. Compared to the isolated cultivation of individual organ models, interaction with the other organs sometimes even improves the MOC’s organ-specific functions. The MOC is connected to an electronic control element that allows computer-based regulation of various parameters such as flow rate or the order in which the organs are actuated.
Four-organ chip
Nutrient solutions can be introduced into the system, but also drugs, chemicals, toxins or even combinations of active substances. Testing can simulate intravenous or oral administration of certain substances. If a particular drug is to be administered orally to the patient, it is first introduced to the MOC’s intestine, where it is first absorbed and then passed on to the other organs such as the liver and kidneys, where it is broken down and excreted. These systems are rapidly taking hold especially in the field of toxicology (toxicity testing), as the data they provide are relevant to humans.
The effect the introduced substances have on the individual organs can be investigated by removing the organ models from the chip and analysing them. The degradation of an active substance can also be tracked by analysing the samples taken from the microchannel system (pharmacokinetic investigation). Components such as a micropump or pH and oxygen sensors are integrated into the chip, making it possible to simulate blood pressure and pulse (42). According to the scientific question, different types and different numbers of organs are placed on the chip. For example, an MOC for diabetes research contains a pancreatic model, as well as insulin-sensitive organs such as the liver or muscle (43), whereas liver, adipose tissue and a blood vessel model are used for research into obesity (44).
Scientists at the Massachusetts Institute of Technology, USA, developed an organ chip, also known as the microphysiological system (MPS), which houses 10 human mini-organs: pancreas, liver, intestines, lungs, heart, muscle, brain, uterus, skin and kidney (45). The mini-organs interact with each other in the MPS, have an actual metabolism and perform functions of the real organs for up to 4 weeks. The well-known anti-inflammatory drug diclofenac was tested in the MPS, with distribution and degradation in the mini-organs and the microfluidic system reflecting the metabolism of the drug in the human body.
The MPS is currently being established for high-throughput applications, automated processes in which thousands of substances can be measured in a very short time. This is crucial for gaining a foothold in the pharmaceutical industry. The cell culture models used are also continuously being developed so that they increasingly resemble real human organs.
Illustration: Holloway PM et al. Stroke 2016 (modified)
MOCs in Space
NASA is funding a research program that will fly MOCs with modern human cell culture systems to the ISS National Lab, a laboratory on the International Space Station (ISS) (46). These MOCs simulate, for example, a human immune system comprising immune cells, bone marrow cells and blood vessel cells. The chips will be tested in space for two weeks, frozen to preserve them, and will then undergo various analyses after their return to Earth. The aim of this research is to ascertain how being in space affects the human immune system’s ageing process, so as to draw conclusions about the body’s own repair mechanisms. A kidney chip will also be sent to the ISS laboratory, as well as bone and cartilage chips, and a chip that models the blood-brain barrier. There will also be a chip on which lungs and bone marrow are connected, for research into inflammation research.
Multi-organ chips will be used to investigate how being in space affects the human immune system’s ageing process of the. (Symbolic image)
Illustration: Pixabay/Doctors Against Animal Experiments e.V.
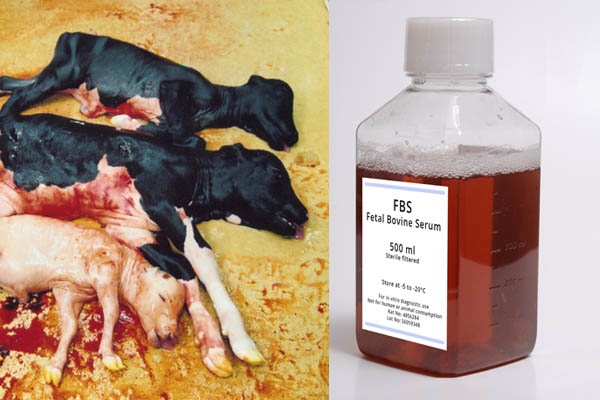
Fetal Calf Serum
Cells need a nutrient medium to stay alive and grow, a liquid containing various nutrients. To date, the default medium used for this has been blood serum from unborn calves. To obtain fetal calf serum (FCS), the fetus is excised from the uterus of a pregnant cow immediately after slaughter. A thick needle is inserted into the beating heart of the calf, which is still alive, and the blood is pumped out until the animal is exsanguinated and dies. This procedure is conducted without anaesthesia, although it can be assumed that calf fetuses are already capable of suffering (47). There are already many FCS-free culture media (48). Human platelet lysate (hPL), for example, is obtained from expired donated blood, which would normally be discarded. In addition, there is a whole range of other FCS-free culture media that are obtained from human blood or produced synthetically. The biggest problem with switching to these ethically sound culture media is that laboratories prefer to fall back on the tried and tested. After all, fetal calf serum has been considered the “gold standard” since the 1960s – despite the fact that FCS’s variable composition can falsify the results.
Video „Mini organs and multi-organ-chips - Scientific progress without animal experiments“
Mit dem Aufruf des Videos erklären Sie sich einverstanden, dass Ihre Daten an YouTube übermittelt werden und das Sie die Datenschutzerklärung gelesen haben.
3. Computer Models
So-called in silico methods are also highly relevant. These are computer-based methods that have been developed in recent years to predict the efficacy and toxicity of substances in the human organism. The chemical properties of new substances are predicted with the help of such methods and can be compared with data from chemicals that have already been approved. Such computer programs have been shown to be better at predicting the toxicity of chemicals than animal experiments (10–12). In silico methods such as QSAR (Quantitative Structure-Activity Relationship) or PBKD (Physiologically Based Kinetic and Dynamic) have already been validated by the European Centre for the Validation of Alternative Methods (ECVAM) and the OECD, and accepted as an alternative to regulatory animal testing (49).
4. Imaging Techniques
Imaging techniques have been around for many decades and they are constantly being developed and optimised. This allows insights in the field of basic human medical research to be gained directly from human patients. These imaging techniques include CT scans and other tomographic procedures such as MRI (50,51), which depict the organs as three-dimensional images. In brain research, individual areas of the human brain can be imaged during certain brain functions. Active brain cells can be identified while a proband memorises images or words or performs other tasks. Such studies of the human brain using imaging techniques provide much better information than absurd behavioural experiments on animals, which often use mice, rats or insects. The imaging techniques are also suitable for studying neurological diseases and diagnosing brain tumours.
A commonly expressed point of criticism regarding human cell culture models or multi-organ chips is the lack of a complete organism for investigating systemic effects. However, if one combines the complex human-based cell models with computer simulations and examinations on probands/patients using modern imaging techniques, one has a comprehensive portfolio for reliable biomedical tests.
Computer tomography and other tomographic imaging methods map organs as three-dimensional images.
Illustration: [Quelle fehlt]
5. Ethically Justifiable Research on Humans
Clinical Trials
There is no doubt that a living human being is the most meaningful object of research for producing data relevant to humans. This is why non-invasive or minimally invasive human studies are invaluable for gaining valuable insights. Of course, high-risk experiments, such as testing a new drug, cannot be carried out directly on humans. There are clinical trials on humans that are implemented as part of drug development before an active ingredient is approved. Before these clinical trials on human subjects start, preclinical pre-tests carried out. These are undoubtedly necessary to provide the best possible protection for the human probands in advance, however in the field of drug development, it is still mandatory that these preclinical tests be carried out on animals. This means that an active substance can only be tested on human probands once it has been found to be safe and effective in animal experiments. This poses a high risk to humans, as animals often react very differently to substances than humans. The fact that animal experiments are not a suitable model for predicting a substance effect in humans is a problem that is increasingly criticised in the scientific world. Numerous studies show that up to 95% of drugs found to be safe and effective in preclinical animal studies fail in human clinical trials (52–54).
Population Studies
Valuable research findings can also be gained with the help of epidemiological studies (population studies), which study groups of people. These can uncover the links between certain diseases and people’s lifestyles and living conditions, such as diet, habits and work. In particular, the combination of epidemiological and epigenetic (the way genes are transcribed) analyses is a field of research that is developing rapidly and provides very valuable insights into human health (55, 56). The results of epidemiological studies allow the development of important preventive measures. Thus, the carcinogenic properties of tobacco smoke and asbestos were recognised. Based on epidemiological studies, it was possible to identify diets rich in meat and fats, lack of exercise and psychosocial factors as the main causes of diabetes, cancer and cardiovascular diseases such as stroke, heart attack and atherosclerosis.
III. Animal-Free Methods in Education and Training
Anyone wanting to become a biologist, biology teacher, doctor or veterinarian will be confronted with animal experiments from the very beginning of their studies at most German universities. This includes using animals or parts of animals killed especially for study purposes, dissecting animals and practising on prepared organs, but experiments on living animals are also intended to teach students basic concepts regarding animals’ structures and their organs’ functions. In zoology internships, for instance, rats, snails, insects and other animals are killed and dissected so as to become acquainted with the appearance and position of the organs. Animal experiments are widespread in physiological studies, especially the notorious experiments on frogs. The frogs’ heads are cut off, then organs such as nerves, muscles or the heart are removed. Even when separated from the body, the organs react to stimuli such as electric shocks or the application of certain drugs. The Italian physician Aloysius Galvani first described the electrical stimulation of frog muscles in 1780, since when generations of students around the world have carried out this experiment on many millions of frogs.
On the other hand, there are at least 1,200 animal-free teaching aids available (57). Many universities have already adopted these innovations, while others still cling to archaic methods.
1. Computer Simulations
Modern computer programs can be used to imitate physiological phenomena in a lifelike way. A curve, for instance stimulus frequency, is not mapped by the twitching frog muscle, but by the computer after the student has set various parameters in the virtual laboratory. Many programs are highly interactive and require the student’s active participation. This achieves a particularly high level of memorisation. There are computer simulations not only for physiological experiments, but also for morphological preparations, pharmacological experiments and many more.
2. Self-Experiments
One can experience physiology with harmless self-experiments on one’s own body. This considerably heightens the learning effect. Myographic tests to determine muscle currents and mechanics can conducted on a student’s arm instead of using a frog muscle.
3. Responsible Use of Dead Animals
There is absolutely no need to kill animals especially for anatomical studies. After all, people are not killed especially for the anatomy courses when studying human medicine. In veterinary clinics and surgeries, there are animals that have died or have been euthanised for medical reasons, which can be used for this purpose. Vertebrate animals that have died natural deaths, or insects and earthworms that have been found dead, are of course also suitable for use by biology students.
4. Plastination
The process of plastination transforms organs or entire animals into a rubber-like state that can be preserved indefinitely without losing shape and colour.
5. Simulators
Lifelike dummies are available for both human and veterinary medicine. The “patients” have a pulse and heartbeat, they breathe and bleed. Students can practise surgical procedures, emergency measures and medical treatment methods on them.
The TraumaMan is an anatomically correct human model that makes it possible to practise a variety of surgical procedures. Artificial blood can be pumped through the “body”, causing the tissue to bleed if a mistake is made. (Illustration: Skils Med Deutschland GmbH)
SynDaver’s true-to-life surgical human model allows for repeated surgical interventions and offers adaptable, variable pathologies with an integrated vascular system. (Illustration: SynDaver)
6. Virtual Reality
Virtual reality for surgeons works like a flight simulator in pilot training. A real-time simulation with video recordings from real surgeries and haptic perception means surgeons feel when they touch tissue with their instruments, cut it, or pull or pushes it with tweezers. The simulation program converts the tactile sensation to display corresponding images on the screen, sourced from a vast video database.
7. Learning from Patients
Veterinary medicine students can learn treatment methods and diagnostic tests (ECG, blood sampling, reflexes, etc.) conducted on animal patients, as is commonplace in human medicine.
8. Assistance
Doctors in training first learn surgical skills by practising on human corpses, veterinarians in training by practising on animals that died of natural causes or were euthanised for medical reasons. The next step is for the doctors in training to assist an experienced surgeon until they are able to perform operations on the patient themselves – initially under supervision. That is a meaningful way to learn the handcraft of surgery.
IV. Validating Animal-Free Testing Methods
Validation is the scientific verification of a research method for its practical application. This involves lengthy and complex procedures to test its reliability, repeatability and ability to predict harmful effects.
Proponents of animal testing often criticise animal-free research methods for not being validated. This is quite simply a falsehood. Many such methods have already successfully been validated. What has never been demonstrably validated, however, are the animal experiments. If the animal-free methods were as bad at predicting results and as poorly reproducible as animal experiments, they would not survive the validation process. Conversely, if animal experiments were subjected to the validation procedures for animal-free methods, they would fail and would not be allowed to be used.
1. Why Are Research Methods Validated?
Validating animal-free test procedures is a major issue when it comes to establishing these methods as “alternatives” to animal testing and accepting them for regulatory (legally required) testing. For it to officially be declared an alternative to animal testing, the animal-free method must be accepted by the European Commission and the Organisation for Economic Cooperation and Development (OECD), an association of the most important industrialised nations. The road to a test procedure’s regulatory acceptance is long and rocky, involving many defined steps and examination by a number of committees, who decide based on various criteria whether the respective method is suitable (58). Validating the test is only the first step, followed by further stages it must go through before finally being accepted by regulators. Validation itself is a rigorous process that must meet exact criteria set by the European Centre for the Validation of Alternative Methods (ECVAM) and the OECD (59).
Our NAT database (see p. 1) provides the possibility of filtering results according to validated methods. These make up only a fraction of all database entries, which reflects the situation in real life. Most animal-free methods developed worldwide are in fact not validated. They are often used for academic or basic research, in which the focus is usually not on the validation of a research method, but on new scientific insights. In this context, research models and methods are simply means for researching scientific facts.
2. Who Is Responsible for Validating Research Methods?
The European Centre for the Validation of Alternative Methods (ECVAM), is responsible for validating alternative methods to animal experiments in Europe (60). Its task is to accompany and coordinate the path of animal-free procedures towards regulatory acceptance, so that they can be used in safety assessment instead of previously mandated animal tests. Analogous institutions in countries outside Europe cooperate closely with ECVAM, and there is also an ongoing exchange with the OECD. This is very important in light of our globalised economy, so that ideally authorities worldwide will accept a test method as an alternative to animal testing, for instance when it a chemical substance is registered or a new product is developed. ECVAM is part of the JRC (Joint Research Centre) and reports to the European Commission.
3. How Does Validation Work?
When an animal-free test method is registered with ECVAM, several committees and groups of stakeholders are consulted before the validation process begins. These assess the relevance of the method. Only then does the extremely complex validation process begin, akin to a quality assurance procedure. The ECVAM specifies specific experimental criteria the test must fulfil. These are strict requirements that demonstrate the reliability of the test procedure and ensure that the test always produces consistent results, regardless of by whom or where it is conducted. This means that the results must display a certain degree of reproducibility or be the same when delivered by different laboratories.
The validation studies are usually carried out in ECVAM’s own laboratories or in the so-called NETVAL laboratories (Network of Laboratories for the Validation of Alternative Methods), about forty accredited laboratories at various locations in Europe, including Germany, that cooperate with ECVAM (61). After validation, the results are reviewed by the ECVAM Scientific Advisory Committee (ESAC). Finally, ECVAM, in cooperation with other bodies of stakeholders and experts, prepares a recommendation for a method. This recommendation is then published and submitted to the European Commission. It should be noted that this recommendation is not binding and does not entail a statutory requirement, but should be taken into account and adapted by decision-makers and authorities in the Member States.
4. What Problems Are There?
Finally, the last step after successful validation of a method is the official regulatory acceptance of a method as an “alternative” to animal testing. This method can then be used for certain regulatory testing instead of using animal tests, for instance when checking whether a certain chemical irritates the skin. The problem here is that although the competent European authorities usually accept these tests, animal-free methods that are accepted instead of animal experiments do not exist for all the numerous test criteria to be examined. Further establishment work is therefore needed to completely ban testing on animals.
The entire validation process leading up to official recognition and inclusion in legislation takes several years. Unfortunately, even validated and recognized non-animal methods are often conducted parallel to animal experiments, such as the pyrogen test (62) (see box).
Pyrogen test
For more than 100 years, the standard procedure for testing medicines, vaccines and other substances for fever-inducing impurities (so-called pyrogens) has been to inject the test substance into rabbits to detect possible fever reactions. To do so, the animals are immobilised. As early as the 1990s, scientists at the University of Konstanz in Germany developed an animal-free pyrogen test that works with human blood. This monocyte activation test (MAT) is not only superior to animal testing from an ethical standpoint, but it can also detect a far wider range of contaminants (63). The test was internationally validated in 2005 and included in the European Pharmacopoeia in 2009 – albeit parallel to the rabbit test. The MAT must be validated on a product-by-product basis, which means it must go through a complex verification procedure for each product, whereas this is not required for the rabbit test. This is a drastic example of the double standards applied to the approval of animal-free testing methods. In 2021, the EU authority EDQM announced that it would phase out the rabbit test within 5 years – at last!
For the pyrogen test, rabbits are locked in tight boxes. Photo: unoL-shutterstock.com)
Many animal-free in vitro tests were validated and accepted by regulators when the EU issued a ban on animal testing in the field of cosmetics (64). This ban came into force in 2013 and the situation is a good example of how important clear legal bans on the abolition of animal testing are to promote non-animal research and its regulatory acceptance.
The Failure of the 3Rs
In 1959, the so-called 3R principles were presented by the British researchers William Russell and Rex Burch. The 3Rs stand for:
- Replacement: Animal testing is replaced by an animal-free method.
- Reduction: Instead of conventional animal experiments, a method is used that reduces the number of laboratory animals.
- Refinement: Measures that reduce animal suffering. However, this also includes improved husbandry conditions.
This concept is based on the assumption that tests on animals make sense in principle as a method and does not consider abolishing them. For opponents of animal experimentation, the Rs Reduction and Refinement are out of the question. Even Replacement is only acceptable to a certain extent, as it implies that testing on animals is in principle a suitable method and only needs to be replaced in order to achieve results relevant for humans.
Animal experiments must in fact be rejected not only for ethical reasons, but also because of the lack of transferability to humans. This scientifically critical aspect is not taken into account in the 3R philosophy. Scientific circles also use terms such as “alternative methods” or “substitute and supplementary methods”. Such expressions are also misleading, since animal-free methods are not just substitutes, but actually good science.
5. What Validated Research Methods Already Exist?
Many tests were validated as part of the EU ban on animal testing for cosmetic products, especially tests using human 3D cell culture models or tissue models. These include three-dimensional models of human skin (65), the eye (66) and the respiratory tract (67). In these tests, the complex cell models are sited on a cell culture plate and the substance to be tested just needs to added. In most cases, a photometer detects a colour reaction, the intensity of which reflects the extent to which the test substance damages the cells. For all these models there are now also approved regulatory tests that can be used to determine required test criteria such as eye irritation, skin irritation, irritation of the respiratory tract, etc., without having to use animal experiments.
A detailed overview of all test methods that have already been accepted or are currently in the validation process can be found in the TSAR (Tracking System for Alternative Methods towards Regulatory Acceptance) database (68). However, caution is also advisable here, because not all of these methods are actually free of animal tissues or components, but rather are simply guaranteed not to be tested on living animals.
Especially in the area of safety testing for chemicals, the computer-based (in silico) methods must be emphasised (49). They have established themselves as validated and accepted regulatory “alternative” methods to animal experiments and are now frequently used, for example for approving chemicals or assessing whether the substance could have harmful effects on the human organism.
6. What Needs to Be Improved in the Future?
Despite the increasing number of validated methods that facilitate animal-free testing, validation is unfortunately subject to double standards. Animal testing is still the measure of all things in the regulatory field, the so-called gold standard, and to make things worse, human-based animal-free methods must be measured against it. This is even though it is well-known and has been scientifically proven time and again that the predictive power of animal experiments is poor – even in safety tests and toxicological investigations. Why should an innovative test procedure based on human cells, which thus logically better reflects the situation in humans, have to prove its suitability in animal experiments? This is absurd and also a dilemma in strategic considerations for the abolition of regulatory animal tests. This is why we do not speak of “alternatives” and why we put the term in quotation marks in this article, because an “alternative” implies that something equivalent is being replaced. However, human-based methods are not equivalent, but better.
There needs to be a general rethinking, a departure from the classic “replacement” of prescribed animal experiments, because that’s exactly what won’t work. According to the European Chemicals Agency (ECHA), certain animal experiments can now be “replaced” by alternative methods quite well, even completely, but this is not yet possible for more complex studies (69). One example cited is the difficulty simulating in vitro a 90-day toxicity test on a rat. This is precisely the wrong approach. The goal must not be to simulate in vitro a currently used demonstrably unreliable rat test, but to keep in mind that one wants to investigate the toxic long-term potential of a substance in humans. Who says that the classic 90-day test, in which the rat is administered a potentially toxic substance orally via a throat tube every day(!), is the method of choice? Also, this test has never been validated, but simply gained a foothold and has now been carried out as a standard for decades. If this method were to be validated correctly, it would most likely fail, because the susceptibility of the test to errors and its lack of transferability to humans is undeniable.
What Needs to Be Done
Unfortunately, neither the EU nor Germany have yet developed a concrete strategy to completely replace animal testing (at least regulatory tests) with animal-free methods. However, it is good and pleasing that there is open and critical discussion with all stakeholders, including NGOs, European authorities and decision-makers. Together with our European umbrella organisations ECEAE (European Coalition to End Animal Experiments) and Eurogroup for Animals, we also highlight the need for action to develop concrete ideas and strategies so that decision-makers at national and European levels focus clearly on abolishing animal experiments. This includes two things in particular: giving the innovative non-animal methods the trust they deserve; and not looking away from the fact that animal experiments with their lack of meaningful results must not be allowed to be the research model for the future.
7. Are Animal-Free Methods Sufficiently Funded?
There is in fact barely any government funding in Germany for the urgently needed validation of animal-free methods. In most cases, it is companies that invest large sums of money in validation when they are interested in a particular method. The pharmaceutical industry could save up to 600 million euros in the development of a single drug if the new processes were used (70). How much money is invested in animal-free research is not publicly communicated by state funding agencies and research associations. Detailed research shows that less than 1% of government funding in research is used for animal-free methods, and over 99% still goes to projects that involve animal testing (71). As long as there is no drastic change in this imbalance, establishing animal-free research in Germany to the extent needed will remain difficult.
V. Paradigm Shift towards Animal-Free Research
Animal-free research has a hard time asserting itself, not least because animal experiments have been deeply seated in society for decades. The image of irreplaceable animal experiments essential for human safety is stubbornly upheld, and fears are stirred up in the population. Scientifically sound evidence that clearly debunks this picture is ignored and hushed up. That is why it is so important to educate and to dispel unfounded dogmas, in order to achieve a paradigm shift towards animal-free science and research.
1. Why Are Animal Experiments Still Being Carried out at All?
If animal experiments deliver such poor results and animal-free research is so superior, why do so many animals still have to die in experiments?
- For more than 150 years, animal experimentation has been regarded as the “gold standard” method in science, without its medical and scientific relevance ever having been tested or validated.
- Huge sums of money flow into research using animals, in the form of research funding, third-party funding or scholarships.
- It’s only possible to make a name for yourself in the world of science if you have a long list of publications in prominent journals. These often require animal testing.
- Around half of all animal experiments are carried out in the context of basic research. This is curiosity-driven research without any direct connection to medical necessity or targeted applications.
- About a quarter of animal experiments are currently required by law for safety testing and drug development.
2. Do Animals Really Mirror Humans?
Animal testing is not an effective research model for researching human diseases or predicting the effect of substances on the human body. Animals’ physiology differs greatly to that of humans, and they react differently to substances than humans. Due to the lack of transferability of experimental results from animals to humans, animal experimental data is inherently unreliable and poses a safety risk to humans. Animals would not naturally develop many of the human diseases that are researched on them, for instance diabetes, Alzheimer’s, Parkinson’s or many forms of cancer. This is why absurd animal models are created by using simple genetic modifications or absurd experimental setups to simulate diseases in healthy animals. Such approaches are bound to fail, since complex human diseases which develop over periods of years or decades and involve many influencing factors cannot be mapped by highly simplified artificially induced changes in animals.
It is therefore absolutely necessary to establish new animal-free models that are human-based and provide valid data in order to make medical research more reliable and efficient.
3. Would There Be no Medical Progress without Animal Testing?
Proponents of animal testing often point out that animal experiments have been involved in the development of numerous drugs. Since successfully completed animal experiments are the prerequisite for a drug to be able to continue through the approval process at all, they are inevitably involved in its development. However, this does not mean that the drug could not have been developed without animal testing. In addition, animal experiments are even a hindrance because they steer research in the wrong direction. Studies show that up to 95% of drugs that have been shown to be safe and effective in animal studies do not reach the market (52–54). The main reason for this is that the active substances sometimes have severe or fatal side effects in the clinical trials on humans, or simply do not work as hoped (72–75). In addition, there is the risk that many drugs that might be effective and unproblematic in humans will be eliminated in animal experiments because of a lack of efficacy or undesirable side effects. Many important drugs such as aspirin, ibuprofen or penicillin would have been withheld from us if we had relied on animal experiments in earlier times. These substances cause serious harm in certain animal species due to different metabolic processes. When these drugs were discovered a long time ago, there was no mandatory testing on animals. They would have failed if subjected to today’s approach to finding pharmaceutical drugs.
Switching to animal-free research methods would also be economically viable, as more and more studies are showing (4,7,76), because the modern methods are cheaper and quicker than lengthy animal experiments. They are also have a high throughput, allowing parallel testing of substances in large quantities.
If modern human-based models were used instead of animal experiments, medical progress would not come to a standstill, but would definitely experience a significant upturn.
25/05/2023
Dr Tamara Zietek
Sources
- Pound P., Bracken M.B. Is animal research sufficiently evidence based to be a cornerstone of biomedical research? BMJ 2014; 348:g3387–g3387
- Pound P. et al. Where is the evidence that animal research benefits humans? BMJ. 2004; 328(7438):514–7
- van der Worp H.B. et al. Can Animal Models of Disease Reliably Inform Human Studies? PLoS Medicine. 2010; 7(3):e1000245
- Meigs L. et al. Animal testing and its alternatives – the most important omics is economics 2018; 35(3):275–305
- New approach methods work plan: Reducing use of animals in chemical testing. USEPA 2020
- Netherlands national Committee for the Protection of animals used for scientific purposes: NCad opinion Transition to non-animal research. 2016
- A non-animal technologies roadmap for the UK: Advancing predicitive biology. 2015
- Eurogroup for animals: Norwegian National Experimental Animals Committee proposes steps to develop a concrete plan for a transition to non-animal science. News. 2020
- Ärzte gegen Tierversuche e.V.: Förderung von Tierversuchen und tierversuchsfreier Forschung. 03.05.2021
- Hartung T. Predicting toxicity of chemicals: software beats animal testing. EFSA Journal 2019; 17(S1):e170710
- Noorden R.V. Software beats animal tests at predicting toxicity of chemicals. Nature 2018; 559:163
- Passini E. et al. Human in silico drug trials demonstrate higher accuracy than animal models in predicting clinical pro-arrhythmic cardiotoxicity. Front Physiol 2017; 8:668
- Sato T. et al. Long-term expansion of epithelial organoids from human colon, adenoma, adenocarcinoma, and Barrett’s epithelium. Gastroenterology. 2011; 141(5):1762–72
- Spence J.R. et al. Directed differentiation of human pluripotent stem cells into intestinal tissue in vitro. Nature 2011; 470(7332):105–9
- Lancaster M.A. et al. Cerebral organoids model human brain development and microcephaly. Nature 2013; 501(7467):373–9
- Takebe T., Wells J.M. Organoids by design. Science 2019; 364(6444):956–9
- Mithal A. et al. Generation of mesenchyme free intestinal organoids from human induced pluripotent stem cells. Nat Commun 2020; 11(1):1–15
- van de Wetering M. et al. Prospective derivation of a Living Organoid Biobank of colorectal cancer patients. Cell 2015; 161(4):933–45
- Schwank G. et al. Functional repair of CFTR by CRISPR/Cas9 in Intestinal Stem Cell Organoids of Cystic Fibrosis Patients. Cell Stem Cell 2013; 13(6):653–8
- Fujii M. et al. Modeling human digestive diseases with CRISPR-Cas9-modified organoids. Gastroenterology 2019; 156(3):562–76
- Clevers H. Modeling Development and Disease with Organoids. Cell 2016; 165(7):1586–97
- Tcw J. Human iPSC application in Alzheimer’s disease and Tau-related neurodegenerative diseases. Neurosci Lett 2019; 23(699):31–40
- Burbulla L.F. et al. Dopamine oxidation mediates mitochondrial and lysosomal dysfunction in Parkinson’s disease. Science 2017; 357(6357):1255–61
- Noh H. et al. Modeling schizophrenia pathogenesis using patient-derived induced pluripotent stem cells (iPSCs). Biochim Biophys Acta 2017; 1863(9):2382–7
- Grunwald L.-M. et al. Comparative characterization of human induced pluripotent stem cells (hiPSC) derived from patients with schizophrenia and autism. Translational Psychiatry 2019; 9(179):1–11
- Victor M.B. et al. Striatal neurons directly converted from Huntington’s disease patient fibroblasts recapitulate age-associated disease phenotypes. Nat Neurosci 2018; 21(3):341–52
- Veening-Griffioen D.H. et al. Are some animal models more equal than others? A case study on the translational value of animal models of efficacy for Alzheimer’s disease. European Journal of Pharmacology 2019; 859:172524
- Cummings J.L. et al. Alzheimer’s disease drug-development pipeline: few candidates, frequent failures. Alzheimers Res Ther 2014; 6(4):37
- Cummings J. Lessons Learned from Alzheimer Disease: Clinical Trials with Negative Outcomes. Clin Transl Sci 2018; 11(2):147–52
- Negraes P.D. et al. Modeling anorexia nervosa: transcriptional insights from human iPSC-derived neurons. Transl Psychiatry 2017; 7(3):e1060
- Plummer S. et al. A Human iPSC-derived 3D platform using primary brain cancer cells to study drug development and personalized medicine. Nature Scientific Reports 2019; 9(1407)
- Halfter K., Mayer B. Bringing 3D tumor models to the clinic - predictive value for personalized medicine. Biotechnol J 2017; 12(2)
- Tuveson D., Clevers H. Cancer modeling meets human organoid technology. Science 2019; 364(6444):952–5
- Ooft S.N. et al. Patient-derived organoids can predict response to chemotherapy in metastatic colorectal cancer patients. Sci Transl Med 2019; 11(513):e2574
- Kim M., Mun H. et al. Patient-derived lung cancer organoids as in vitro cancer models for therapeutic screening. Nat Commun 2019; 10(1):1–15
- Ng W.L. et al. Skin Bioprinting: Impending Reality or Fantasy? Trends Biotechnol 2016; 34(9):689–99
- Berg J., Kurreck J. Clean bioprinting - Fabrication of 3D organ models devoid of animal components. ALTEX 2021; 38(2)
- Anas Essop. Korean bioprinting startup develops 3D respiratory epithelium model for virus research. 3D Printing Industry 2020
- Lansdowne L.E. Using Bioprinted Tissue Models To Test Drugs Against Diseases Including COVID-19. Drug Discovery from Technology Networks 2020
- Augustine R. Skin bioprinting: a novel approach for creating artificial skin from synthetic and natural building blocks. Prog Biomater 2018; 7(2):77–92
- Park S.E. et al. Organoids-on-a-chip. Science 2019; 364(6444):960–5
- Kolanowski T.J. et al. Enhanced structural maturation of human induced pluripotent stem cell-derived cardiomyocytes under a controlled microenvironment in a microfluidic system. Acta Biomater 2020; 102:273–86
- Bauer S. et al. Functional coupling of human pancreatic islets and liver spheroids on-a-chip: Towards a novel human ex vivo type 2 diabetes model. Sci Rep 2017; 7(1):1–11
- Rogal J. et al. Stem-cell based organ-on-a-chip models for diabetes research. Advanced Drug Delivery Reviews. 2019; 140:101–28
- Edington C.D. et al. Interconnected Microphysiological Systems for Quantitative Biology and Pharmacology Studies. Sci Rep 2018; 8(1):4530.
- Johnson M. Tissue Chips in Space a Big Leap for Research. NASA. 9.11.2018
- More S..et al. Animal welfare aspects in respect of the slaughter or killing of pregnant livestock animals (cattle, pigs, sheep, goats, horses). EFSA AHAW 2017; 15(5):55
- Fetal Calf Serum Free Database. https://fcs-free.sites.uu.nl/ (abgerufen am 4.5.2021)
- Dura A. Alternative methods for toxicity testing: Computational Methods. EU Science Hub - European Commission 2018
- Touska P., Connor S.E.J. Recent advances in MRI of the head and neck, skull base and cranial nerves: new and evolving sequences, analyses and clinical applications. Br J Radiol 2019; 92(1104):20190513
- Aziz W. et al. Emerging role of cardiac computed tomography in heart failure. ESC Heart Fail 2019; 6(5):909–20
- Thomas D.W. et al. Clinical Development Success Rates 2006-2015. BIO Industry Analysis. June 2016
- Mullard A. Parsing clinical success rates. Nat Rev Drug Discov 2016; 15(447)
- Wong C.H. et al. Estimation of clinical trial success rates and related parameters. Biostatistics 2019; 20(2):273–86
- Mill J., Heijmans B.T. From promises to practical strategies in epigenetic epidemiology. Nat Rev Genet 2013; 14(8):585–94
- Bakulski K.M., Fallin M.D. Epigenetic epidemiology: promises for public health research. Environ Mol Mutagen. 2014; 55(3):171–83
- InterNICHE-Alternativendatenbank. https://interniche.org/de/alternatives (abgerufen am 4.5.2021)
- Bingham M. Validation and submission process. EU Science Hub - European Commission 2017
- Guidance document on the validation and international acceptance of new or updated test methods for hazard assessment. OECD 2005; 34
- O’Connor L. EU Reference Laboratory for alternatives to animal testing. EU Science Hub - European Commission 2017
- Bingham M. EU-NETVAL (European Union Network of Laboratories for the Validation of Alternative Methods). EU Science Hub - European Commission 2017
- Gericke C. Pyrogentest - Unendlich viel Tierleid trotz vorhandener tierversuchsfreier Methoden. Ärzte gegen Tierversuche e.V., 06.07.2020
- Hartung T. The human whole blood pyrogen test - lessons learned in twenty years. ALTEX. 2015; 32(2):79–100
- Ärzte gegen Tierversuche e.V. Aus für Kosmetik-Tierversuche! 12.03.2013
- SkinEthic RHE Reconstructed Human Epidermis. https://www.episkin.com/SkinEthic-RHE (abgerufen am 4.5.2021)
- EpiOcular in vitro 3D Tissues. MatTek Life Sciences
- Epithelix. Products mucilair
- EURL ECVAM. Tracking System for Alternative methods towards Regulatory acceptance (TSAR)
- ECHA. The use of alternatives to testing on animals for REACH. 4.11.2020
- Franzen N. et al. Impact of organ-on-a-chip technology on pharmaceutical R&D costs. ScienceDirect 2019; 24(9)
- Ärzte gegen Tierversuche e.V. Förderung von Tierversuchen und tierversuchsfreier Forschung. 3.5.2021
- Cook D. et al. Lessons learned from the fate of AstraZeneca’s drug pipeline: a five-dimensional framework. Nat Rev Drug Discov 2014; 13(6):419–31
- Arrowsmith J. A decade of change. Nat Rev Drug Discov 2012; 11(17-8)
- Waring M.J. et al. An analysis of the attrition of drug candidates from four major pharmaceutical companies. Nat Rev Drug Discov. 2015; 14(7):475–86
- Harrison R.K. Phase II and phase III failures: 2013–2015. Nat Rev Drug Discov 2016;15(817-8)
- Franzen N. et al. Impact of organ-on-a-chip technology on pharmaceutical R&D costs. Drug Discovery Today 2019; 24(9):1720–4